DC Tolerance
General features of dendritic cells (DCs)
General features of dendritic cells (DCs)
DCs have been first described in 1973 by Ralph M. Steinman (1). For his finding he received the Nobel Price in 2011. DCs are well established as antigen presenting cells that can initiate CD4+ and CD8+ T cell immune responses against pathogens (2). They are specialized for this by their capacity to recognize and capture pathogens or their products during their immature stage at the infection site and transport them to the draining lymph nodes (3). There the processed pathogen proteins will be presented by the mature stage on MHC II or cross-presented on MHC I molecules to CD4+ or CD8+ T cells in an immunogenic context (also providing costimulation and proinflammatory cytokines) (4, 5). This old model on general DC biology is still valid but many more aspects of DC biology have been added in the meantime.
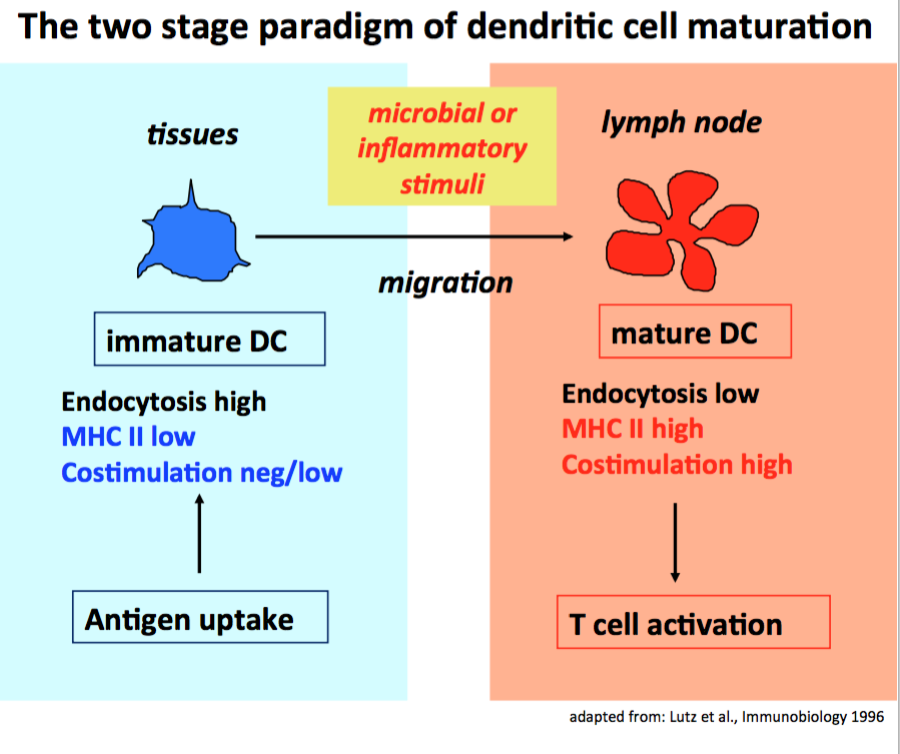
It is also well established but less known that DCs also present glycolipids on CD1a,b,c,d (human) or CD1d (mouse) molecules to invariant NKT cells (iNKT). We found, that the quality of DC maturation can instruct iNKT cells to produce selectively IFN-g or IL-4, similar to Th1 or Th2 cell polarization (6). In addition, DCs can transport protein antigens in their native form in storage compartments at neutral pH (7) for "presentation" to B cells in the lymph nodes (8).
DC subsets
Especially in recent years many researchers reported on the identification of DC subsets. Despite the question, whether DCs should be defined by their developmental origin or their functions (9, 10), there is increasing consent about the number of subsets. Under steady state conditions the growth factors IL-34 and Flt3L generate two subsets of conventional DCs (cDC1 and cDC2) and plasmacytoid DCs (pDCs). While cDC1s are specialized on antigen presentation to CD8+ T cells, the cDC2 subset stimulates better the CD4+ T cells. The subset of pDCs is the major cell type for type I IFN production, thus, relevant for anti-viral defenses (11-13). During inflammatory conditions of infections, monocytes can also develop into functional DCs (MoDCs) to take over functions of both cDC1 and cDC2 (10). While the cDC2 have been further characterized how they functionally polarize CD4+ T cells into T helper cell (Th) subsets, we concentrated on the functions how murine MoDCs induce different CD4+ Th subsets that can be generated from bone marrow with GM-CSF. This is why they are also called BM-DCs. BM-DCs can induce T cell anergy, Tregs (Foxp3+ and Tr1) and polarize into Th1, Th2 and Th17 responses, depending on their maturation/activation state (14, 15).
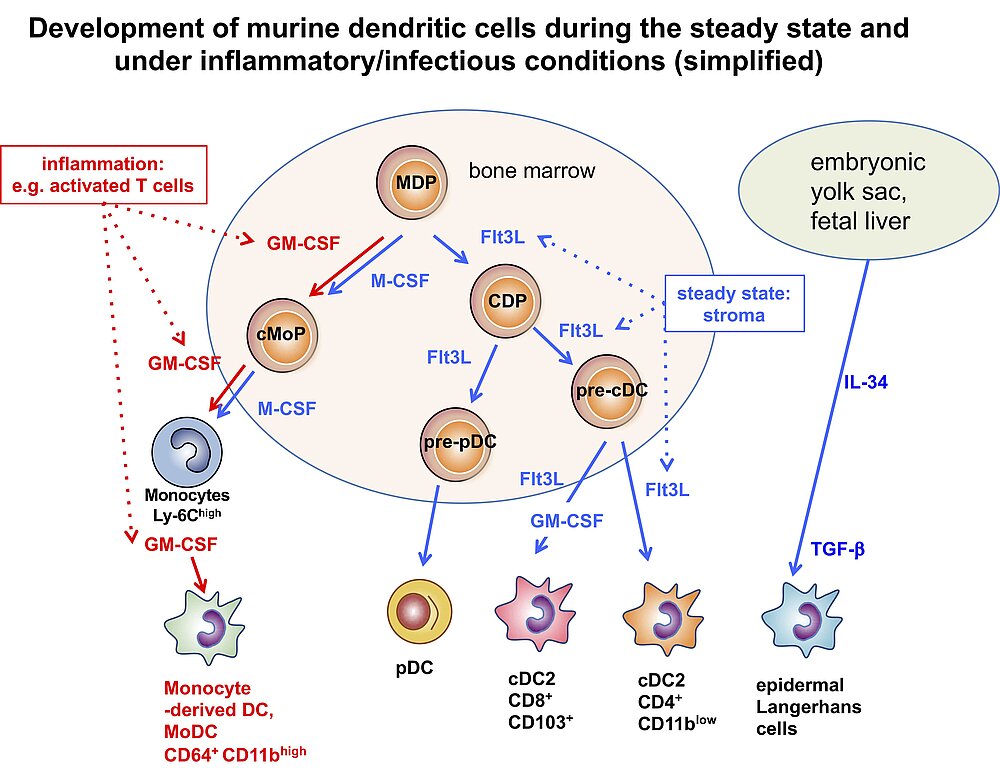
Besides immunogenic functions it became also clear during the last years that immature DCs are not only resting cells waiting for pathogens, but that immature and semi-mature DC maturation stages (see below) are constantly involved to mediate immune tolerance to peripheral antigens (16, 17). These constitutively active DC-mediated peripheral tolerance mechanisms help to avoid autoimmunity in healthy individuals. In addition, they can be used for therapy to revert transplant rejections or to treat autoimmune diseases, allergy and overshooting immune responses against pathogens (18-21).
One of our major research interests is to understand the tolerogenic activities of DCs in healthy individuals and the underlying molecular mechanisms. The methods used are various gene-deficient mice, gene expression profiling and cellular therapy by adoptive transfered DCs into murine disease models. We also try to extend our findings to therapeutic interventions in horses (22) and humans (23, 24).
Research Interests
1. Tolerogenic BM-DC maturation stages (immature & resting, semi-mature & steady state migratory)
Tolerogenicity of DCs is mainly directed by their maturation stage. For every major subset in the mouse (CD8a+, CD4+, monocyte-derived BM-DCs, plasmacytoid DCs) immature stages have been shown to act tolerogenic on T cells and only after maturation convert to immunogenic cells (14).
Earlier we found that BM-DC maturation can lead to semi-mature or fully mature stages. They are distinguishable mainly by their presence or absence of proinflammatory cytokine production, while the expression of MHC II- and costimulatory molecules as well as the expression of the CCR7 homing receptor remain similar. Such semi-mature DCs appeared tolerogenic in a Th1/Th17-driven autoimmune model (EAE)(25) but had no effect in Th2 models (asthma, BALB/c-Leishmania infection)(26) and could even induce autoimmunity of CD8+ T cells in a Type I diabestes model (27). As mechansims we identified Th2- and NKT-cell-mediated immune deviation and IL-10-producing regulatory Tr1 cells.
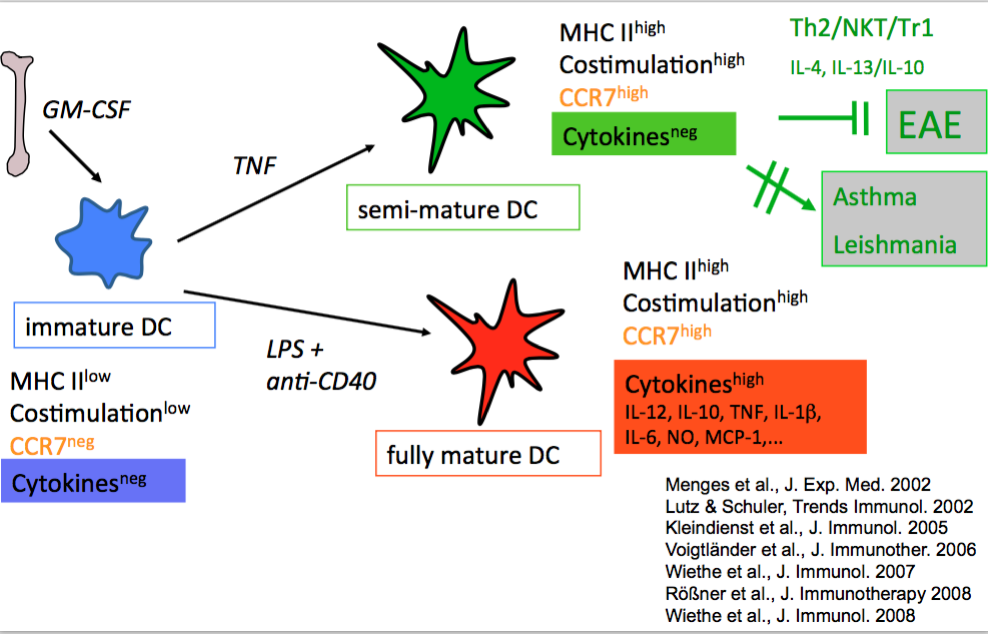
2. Instruction of tolerogenic CD4+ T cell phenotypes by dendritic cells
2.1 T cell anergy
Anergy can be induced by immature DCs in the absence of TGF-b. In this way low level antigen presentation occurs on MHC II molecules (Signal 1) with absent to low costimulation (Signal 2). Anergy induction represents a tolerance mechanism to functionally inactivate T cells for further antigen-specific T cell receptor activation (14, 28). Induction of T cell anergy can be achieved by injection of high doses od antigen in vivo. However, the underlying DC subset for this phenomenon has not been identified yet.
2.2 Regulatory T cells
Regulatory T cells emerge either directly from the thymus (tTregs, or natural Tregs, nTregs) or can be induced in peripheral lymphoid organs (29). In the murine spleen immature DCs of the CD4+ or CD8a+ can convert naive CD4+ T cells into Foxp3+ peripherally induced Tregs (iTregs or pTregs) if TGF-b is present (30). Most likely the capture of soluble self-antigens initiates this process in lymph nodes (31). In addition Foxp3- IL-10+ Tregs, called Tr1, can be induced upon repetitive stimulation of Th1 or Th2 cells or by stimulation of naive T cells in the presence of IL-10 (14, 32, 33).
In peripheral lymph nodes steady state migratory DCs constitutively transport tissue-associated self-antigens from peripheral tissues to the draining lymph node (34). They appear at a semi-mature stage (16), e.g. express RelB/p52 but not RelA/p50 as compared with fully mature/immunogenic DCs. They also express the lymph node homing receptor CCR7 at their surface which enables their migration into the paracortical T cell areas of lymph nodes. The Langerin+ CD103+ dermal cDC1 carry LAP/TGF-b complexes on their surface leading to Foxp3+ iTreg conversion (35). In contrast, self-antigen-specific CD8+ T cells are deleted (36), most likely by epidermal Langerhans cells (10). More recently, we found that the dermal CD11b+ steady state migratory cDC2 subset helps to maintain the pool of peripherally circulating nTregs in collaboration with self-antigen specific CD4+ Tmem-like cells producing IL-2 for nTreg division (37).
Steady state functions of lymph node DC subsets in mice
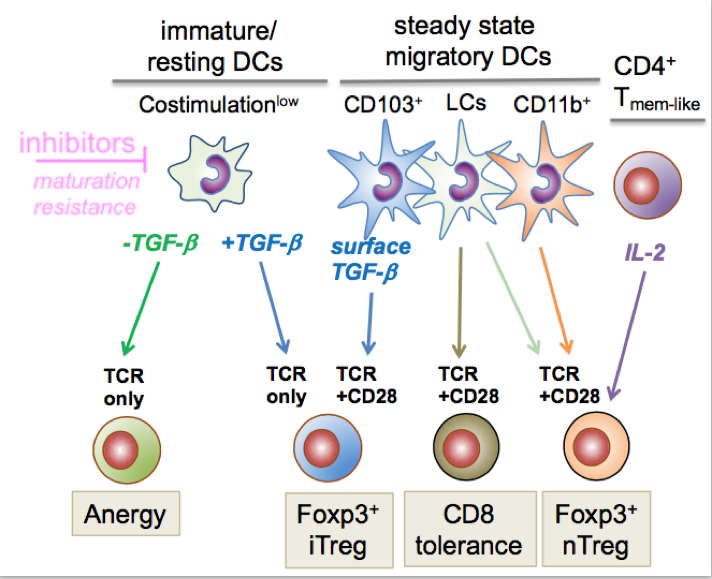
2.3 Polarization of CD4+ T helper cell responses by BM-DCs (as a model for MoDCs)
DCs are not only engaged to prime T cell responses via signal 1 and 2 (see above) but also contribute to further differentiation of T cells (signal 3)(38). For the CD4+ T cell subset this process had been termed polarization into T helper cell (Th) subsets (39, 40).
We are especially interested in the question which pathogen-, danger- or inflammatory signals and signaling receptors instruct BM-DCs for further instruction of T cell polarization into specific Th1, Th2, Th17 or CD4+ regulatory T cell subtypes.
We found that Th2 instruction potential by BM-DCs is characterized by semi-maturation and reflected by a genetic pattern (detected by mRNA microarrays) that encompasses only an inflammatory signature but no specific instructive signals. In this respect TNFa and Trypanosoma brucei antigens were highly overlapping. In contrast, the DC maturation by LPS leading to Th1 instruction induces the same inflammatory plus many more additional genes (full maturation), including IL-12 (41). These data indicate that quantitative differences in DC maturation can direct Th1/Th2 polarization (42).
The gene pattern induced by cholera toxin shows almost no qualitative overlap with the Th-1 instructing LPS or Th2-instructing TNFa or Trypanosoma stimuli. Instead, cholera toxin matured BM-DCs polarize towards Th17 responses (43). Interestingly, low doses of cholera toxin induce CTLA-2a and CTLA-2b molecules in BM-DCs that contribute to Treg conversion in cooperation with TGF-b (43).
This represents one of many examples how pathogens have evolved immune evasion mechanisms at the level of DCs by inducing the expression of tolerogenic molecules (44).
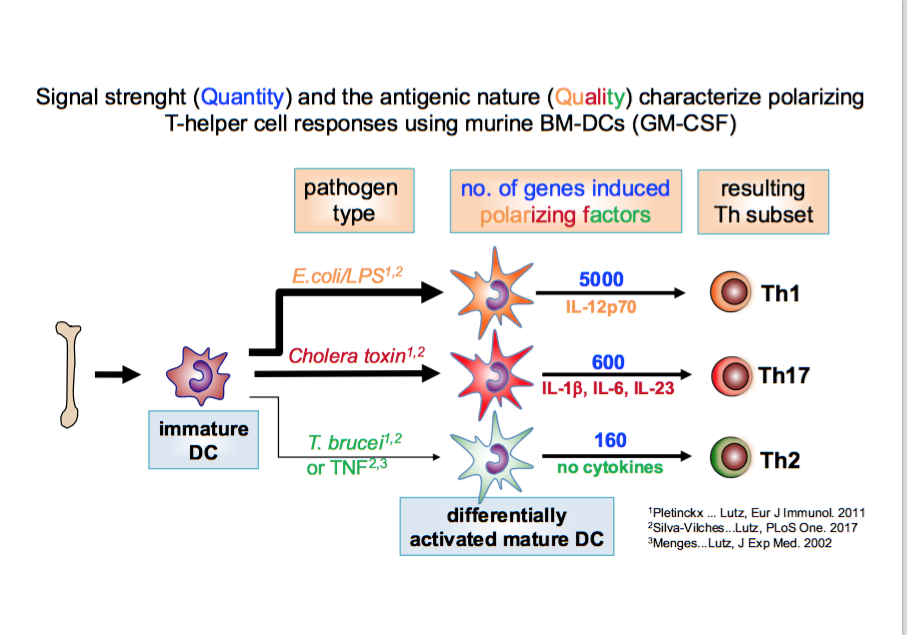
References
1. Steinman, R. M., and Z. A. Cohn. 1973. Identification of a novel cell type in peripheral lymphoid organs of mice. I. Morphology, quantitation, tissue distribution. J. Exp. Med. 137: 1142-1162.
2. Banchereau, J., and R. M. Steinman. 1998. Dendritic cells and the control of immunity. Nature 392: 245-252.
3. Austyn, J. M. 1992. Antigen uptake and presentation by dendritic leukocytes. Seminars in immunology 4: 227-236.
4. Sallusto, F., and A. Lanzavecchia. 1994. Efficient presentation of soluble antigen by cultured human dendritic cells is maintained by granulocyte/macrophage colony-stimulating factor plus interleukin 4 and downregulated by tumor necrosis factor alpha. J Exp Med 179: 1109-1118.
5. Kurts, C., M. Cannarile, I. Klebba, and T. Brocker. 2001. Dendritic cells are sufficient to cross-present self-antigens to CD8 T cells in vivo. Journal of immunology 166: 1439-1442.
6. Wiethe, C., M. Schiemann, D. Busch, L. Haeberle, M. Kopf, G. Schuler, and M. B. Lutz. 2007. Interdependency of MHC class II/self-peptide and CD1d/self-glycolipid presentation by TNF-matured dendritic cells for protection from autoimmunity. Journal of immunology 178: 4908-4916.
7. Lutz, M. B., P. Rovere, M. J. Kleijmeer, M. Rescigno, C. U. Assmann, V. M. Oorschot, H. J. Geuze, J. Trucy, D. Demandolx, J. Davoust, and P. Ricciardi-Castagnoli. 1997. Intracellular routes and selective retention of antigens in mildly acidic cathepsin D/lysosome-associated membrane protein-1/MHC class II-positive vesicles in immature dendritic cells. J. Immunol. 159: 3707-3716.
8. Qi, H., J. G. Egen, A. Y. Huang, and R. N. Germain. 2006. Extrafollicular activation of lymph node B cells by antigen-bearing dendritic cells. Science 312: 1672-1676.
9. Ginhoux, F., M. Guilliams, and S. H. Naik. 2016. Editorial: Dendritic Cell and Macrophage Nomenclature and Classification. Front Immunol 7: 168.
10. Lutz, M. B., H. Strobl, G. Schuler, and N. Romani. 2017. GM-CSF Monocyte-Derived Cells and Langerhans Cells As Part of the Dendritic Cell Family. Front Immunol 8: 1388.
11. Waskow, C., K. Liu, G. Darrasse-Jeze, P. Guermonprez, F. Ginhoux, M. Merad, T. Shengelia, K. Yao, and M. Nussenzweig. 2008. The receptor tyrosine kinase Flt3 is required for dendritic cell development in peripheral lymphoid tissues. Nature immunology 9: 676-683.
12. Merad, M., P. Sathe, J. Helft, J. Miller, and A. Mortha. 2013. The dendritic cell lineage: ontogeny and function of dendritic cells and their subsets in the steady state and the inflamed setting. Annual review of immunology 31: 563-604.
13. See, P., C. A. Dutertre, J. Chen, P. Gunther, N. McGovern, S. E. Irac, M. Gunawan, M. Beyer, K. Handler, K. Duan, H. R. B. Sumatoh, N. Ruffin, M. Jouve, E. Gea-Mallorqui, R. C. M. Hennekam, T. Lim, C. C. Yip, M. Wen, B. Malleret, I. Low, N. B. Shadan, C. F. S. Fen, A. Tay, J. Lum, F. Zolezzi, A. Larbi, M. Poidinger, J. K. Y. Chan, Q. Chen, L. Renia, M. Haniffa, P. Benaroch, A. Schlitzer, J. L. Schultze, E. W. Newell, and F. Ginhoux. 2017. Mapping the human DC lineage through the integration of high-dimensional techniques. Science 356: eaag3009.
14. Pletinckx, K., A. Dohler, V. Pavlovic, and M. B. Lutz. 2011. Role of dendritic cell maturity/costimulation for generation, homeostasis, and suppressive activity of regulatory T cells. Front Immunol 2: 39.
15. Lutz, M. B. 2016. Induction of CD4(+) Regulatory and Polarized Effector/helper T Cells by Dendritic Cells. Immune Netw 16: 13-25.
16. Lutz, M. B., and G. Schuler. 2002. Immature, semi-mature and fully mature dendritic cells: which signals induce tolerance or immunity? Trends Immunol 23: 445-449.
17. Steinman, R. M., and M. C. Nussenzweig. 2002. Avoiding horror autotoxicus: the importance of dendritic cells in peripheral T cell tolerance. Proceedings of the National Academy of Sciences of the United States of America 99: 351-358.
18. Lutz, M. B., and C. Kurts. 2009. Induction of peripheral CD4+ T-cell tolerance and CD8+ T-cell cross-tolerance by dendritic cells. Eur. J. Immunol. 39: 2325-2330.
19. Lutz, M. B. 2012. Therapeutic potential of semi-mature dendritic cells for tolerance induction. Front Immunol 3: 123.
20. Beriou, G., A. Moreau, and M. C. Cuturi. 2012. Tolerogenic dendritic cells: applications for solid organ transplantation. Curr Opin Organ Transplant 17: 42-47.
21. Morelli, A. E., and A. W. Thomson. 2007. Tolerogenic dendritic cells and the quest for transplant tolerance. Nature reviews. Immunology 7: 610-621.
22. Dietze, B., E. Cierpka, M. Schäfer, W. Schill, and M. B. Lutz. 2008. An improved method to generate equine dendritic cells from peripheral blood mononuclear cells: divergent maturation programs by IL-4 and LPS. Immunobiology 213: 751-758.
23. Berger, T. G., H. Schulze-Koops, M. Schäfer, E. Müller, and M. B. Lutz. 2009. Immature and maturation-resistant human dendritic cells generated from bone marrow require two stimulations to induce T cell anergy in vitro. PloS one 4: e6645.
24. Baur, A. S., M. B. Lutz, S. Schierer, L. Beltrame, G. Theiner, E. Zinser, C. Ostalecki, G. Heidkamp, I. Haendle, M. Erdmann, M. Wiesinger, W. Leisgang, S. Gross, A. J. Pommer, E. Kämpgen, D. Dudziak, A. Steinkasserer, D. Cavalieri, B. Schuler-Thurner, and G. Schuler. 2013. Denileukin diftitox (ONTAK) induces a tolerogenic phenotype in dendritic cells and stimulates survival of resting Treg. Blood 122: 2185-2194 (*equal contribution).
25. Menges, M., S. Rossner, C. Voigtlander, H. Schindler, N. A. Kukutsch, C. Bogdan, K. Erb, G. Schuler, and M. B. Lutz. 2002. Repetitive injections of dendritic cells matured with tumor necrosis factor alpha induce antigen-specific protection of mice from autoimmunity. J Exp Med 195: 15-21.
26. Wiethe, C., A. Debus, M. Mohrs, A. Steinkasserer, M. B. Lutz, *,, and A. Gessner. 2008. Dendritic cell differentiation state and their interaction with NKT cells determine Th1/Th2 differentiation in the murine model of Leishmania major infection. Journal of immunology 180: 4371-4381 *equal contribution with last author.
27. Kleindienst, P., C. Wiethe, M. B. Lutz, and T. Brocker. 2005. Simultaneous induction of CD4 T cell tolerance and CD8 T cell immunity by semimature dendritic cells. Journal of immunology 174: 3941-3947.
28. Schwartz, R. H., D. L. Mueller, and M. K. Jenkins. 1989. T-cell clonal anergy. Cold Spring Harbor Symp. Quant. Biol. 54: 605-610.
29. Wing, K., and S. Sakaguchi. 2010. Regulatory T cells exert checks and balances on self tolerance and autoimmunity. Nature immunology 11: 7-13.
30. Yamazaki, S., D. Dudziak, G. F. Heidkamp, C. Fiorese, A. J. Bonito, K. Inaba, M. C. Nussenzweig, and R. M. Steinman. 2008. CD8+ CD205+ splenic dendritic cells are specialized to induce Foxp3+ regulatory T cells. Journal of immunology 181: 6923-6933.
31. Sixt, M., N. Kanazawa, M. Selg, T. Samson, G. Roos, D. P. Reinhardt, R. Pabst, M. B. Lutz, and L. Sorokin. 2005. The conduit system transports soluble antigens from the afferent lymph to resident dendritic cells in the T cell area of the lymph node. Immunity 22: 19-29.
32. Roncarolo, M. G., and M. Battaglia. 2007. Regulatory T-cell immunotherapy for tolerance to self antigens and alloantigens in humans. Nature reviews. Immunology 7: 585-598.
33. O'Garra, A., P. L. Vieira, P. Vieira, and A. E. Goldfeld. 2004. IL-10-producing and naturally occurring CD4+ Tregs: limiting collateral damage. The Journal of clinical investigation 114: 1372-1378.
34. Lutz, M. B., A. Dohler, and H. Azukizawa. 2010. Revisiting the tolerogenicity of epidermal Langerhans cells. Immunology and cell biology 88: 381-386.
35. Azukizawa, H., A. Dohler, N. Kanazawa, A. Nayak, M. Lipp, B. Malissen, I. Autenrieth, I. Katayama, M. Riemann, F. Weih, F. Berberich-Siebelt, and M. B. Lutz. 2011. Steady state migratory RelB+ langerin+ dermal dendritic cells mediate peripheral induction of antigen-specific CD4+ CD25+ Foxp3+ regulatory T cells. Eur. J. Immunol. 41: 1420-1434.
36. Waithman, J., R. S. Allan, H. Kosaka, H. Azukizawa, K. Shortman, M. B. Lutz, W. R. Heath, F. R. Carbone, and G. T. Belz. 2007. Skin-derived dendritic cells can mediate deletional tolerance of class I-restricted self-reactive T cells. J. Immunol. 179: 4535-4541.
37. Dohler, A., T. Schneider, I. Eckert, E. Ribechini, N. Andreas, M. Riemann, B. Reizis, F. Weih, and M. B. Lutz. 2017. RelB+ Steady-State Migratory Dendritic Cells Control the Peripheral Pool of the Natural Foxp3+ Regulatory T Cells. Front Immunol 8: 726.
38. Kalinski, P., C. M. Hilkens, E. A. Wierenga, and M. L. Kapsenberg. 1999. T-cell priming by type-1 and type-2 polarized dendritic cells: the concept of a third signal. Immunol Today 20: 561-567.
39. Moser, M., and K. M. Rock. 2000. Dendritc cell regulation of TH1-TH2 development. Nat. Immunol. 1: 199-205.
40. O'Shea, J. J., and W. E. Paul. 2010. Mechanisms underlying lineage commitment and plasticity of helper CD4+ T cells. Science 327: 1098-1102.
41. Pletinckx, K., B. Stijlemans, V. Pavlovic, R. Laube, C. Brandl, S. Kneitz, A. Beschin, P. De Baetselier, and M. B. Lutz. 2011. Similar inflammatory DC maturation signatures induced by TNF or Trypanosoma brucei antigens instruct default Th2-cell responses. Eur J Immunol 41: 3479-3494.
42. Lutz, M. B. 2013. How quantitative differences in dendritic cell maturation can direct Th1/Th2-cell polarization. Oncoimmunology 2: e22796.
43. Silva-Vilches, C., K. Pletinckx, M. Lohnert, V. Pavlovic, D. Ashour, V. John, E. Vendelova, S. Kneitz, J. Zhou, R. Chen, T. Reinheckel, T. D. Mueller, J. Bodem, and M. B. Lutz. 2017. Low doses of cholera toxin and its mediator cAMP induce CTLA-2 secretion by dendritic cells to enhance regulatory T cell conversion. PloS one 12: e0178114.
44. Vendelova, E., D. Ashour, P. Blank, F. Erhard, A. E. Saliba, U. Kalinke, and M. B. Lutz. 2018. Tolerogenic Transcriptional Signatures of Steady-State and Pathogen-Induced Dendritic Cells. Front Immunol 9: 333.